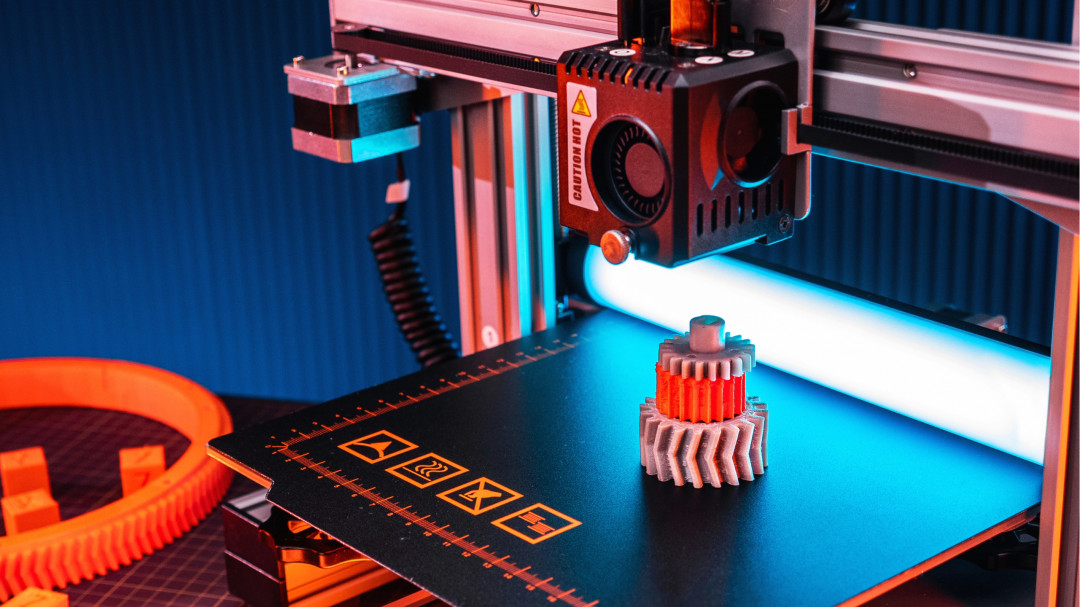
Although the 3D printing process has been in use for more than three decades, the technology is still young compared to established manufacturing techniques. There is still room for improvement, particularly in the starting materials, especially when it comes to metallic materials. However, 3D printing can be used to manufacture products with complex material compositions that were previously impossible, and thus with completely new properties. This is where the newly established field of ‘Materials for Additive Manufacturing’ by Prof. Dr.-Ing. Christian Haase. His professorship is the second TU professorship in cooperation with the Werner-von-Siemens Centre for Industry and Science e.V. (WvSC). This non-profit organisation was established by partners including Siemens AG, TU Berlin, the Fraunhofer-Gesellschaft, the Federal Institute for Materials Research and Testing (BAM) and the State of Berlin in order to better integrate research and value creation. Christian Haase is the recipient of a Starting Grant from the European Research Council (ERC), which has just been launched, and since his appointment he has also become the new scientific director of the Central Unit for 3D Technologies at TU Berlin.
Glowing hot steel flows into an ingot mould and, after solidification, is still glowing when it is rolled into sheets or rods. These can then be deep-drawn, bent or forged into different shapes. ‘Each of these transformations also changes the material structure, although the most important material properties are already determined when the material blank is cast,’ says Christian Haase. In contrast to this top-down approach, additive manufacturing uses a bottom-up method: in 3D printing, the finished product is produced in a single step, except for subsequent work such as polishing. In what is known as the powder bed process, a laser or electron beam selectively melts a material in powder form at specific points, so that a complex workpiece of almost any shape can be created layer by layer. In the case of ‘laser deposition welding’, even completely different materials can be used together to build a workpiece, with the desired material being sprayed on as a powder-gas mixture shortly before it is melted by the laser.
Bottom-up approach in 3D printing
‘This production method allows us to set very different material and surface properties at different points on the workpiece, and at completely different size scales, directly during the forming process,’ says Christian Haase. In addition to the possibility of combining different materials during laser deposition welding, the energy, beam diameter and movement speed of the laser are also parameters that can be used to influence material properties. ‘From the different chemical composition and the arrangement of individual atoms, to larger-scale, desired deviations in the crystal structure, to the grain structure of the material, which is sometimes visible to the naked eye – we can make targeted changes in all these size ranges.’
ERC Starting Grant ‘HeteroGenius4D’
This possibility of integrating not only a practically freely selectable three-dimensional shape into the microstructure of the materials in additive manufacturing, but also completely new material properties, is also referred to in professional circles as the fourth dimension in 3D printing. Over the next five years, Christian Haase will investigate this additional dimension with the ERC Starting Grant ‘HeteroGenius4D’. ‘The difficulty here is that the number of parameters that can be changed is very high. Even the range of chemical compositions that can be worked with is extremely broad, even if you limit yourself to metallic materials,’ explains Haase. Then there are the process parameters such as the properties and guidance of the laser beam. ’So there are a multitude of possible combinations from which you have to filter out the optimum.’
Combining high-throughput experiments and material simulations
To overcome this challenge, Christian Haase relies on computer simulations of new materials that predict their properties. ‘But this only works if these simulations are based on a solid database,’ says Haase. That is why he and his team also conduct high-throughput experiments in which test specimens are created at high speed using laser deposition welding and automated measurements of the hardness of these test specimens and electron microscopic images of them are carried out. ‘In the end, we have entire maps that show how the material properties depend on the chemical composition and, for example, the laser power. On the basis of these maps, simulation programmes can then conduct a refined search for the material properties that are precisely desired for a specific application.’
Applications in aerospace, mobility, the energy transition and the semiconductor industry
3D printing has traditionally been used in industry where complex workpieces are required in small quantities. For example, for casting moulds and special tools in production plants, in the semiconductor industry, but also in the aerospace industry. ‘Additive manufacturing will also play a helpful role in the energy transition,’ says Christian Haase, citing a research project he has carried out in the field of mobility. The aim was to replace the expensive and geopolitically critical element scandium in high-strength aluminium alloys. Using an approach that combined experiments and simulations, Haase's group identified the less expensive element zirconium as a replacement, which showed better properties in the alloy and also saved weight. ‘3D printing can also bring major advantages in the hot areas of gas turbines, whether in aircraft or in the conversion of natural gas or hydrogen into electricity. This is because new geometries make it possible to integrate completely different cooling systems into the turbine,’ says Haase, who also has relevant project experience in this area.